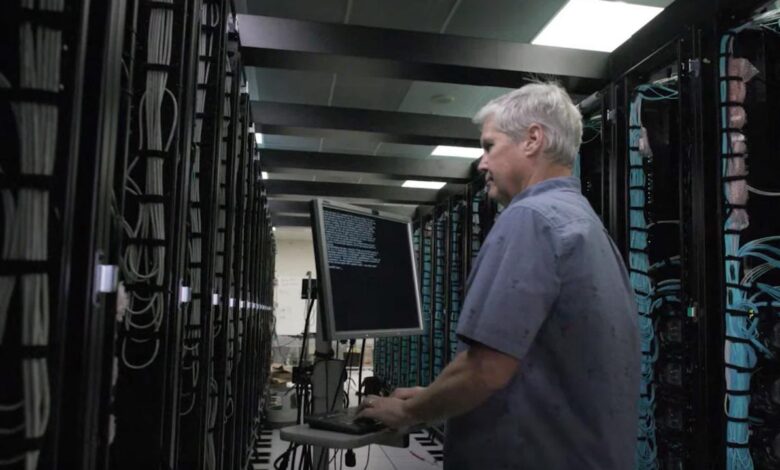
97 Million Supercomputer in Full Swing in Exeter
97 million supercomputer in full swing in Exeter! That’s right, a massive computing powerhouse is now operational, promising breakthroughs in various scientific fields. This incredible machine isn’t just a collection of hardware; it’s a gateway to unlocking answers to some of humanity’s most pressing questions, from climate change modeling to revolutionary drug discoveries. Prepare to be amazed by the sheer scale and potential of this technological marvel.
Imagine a machine with the processing power to simulate entire ecosystems, design groundbreaking new materials, or analyze vast datasets that were previously impossible to tackle. That’s the reality we’re facing with the Exeter supercomputer. Its advanced architecture, featuring interconnected nodes working in perfect harmony, allows for unprecedented speed and efficiency in complex calculations. This isn’t just about raw power; it’s about the potential for real-world impact across a multitude of disciplines.
The Exeter Supercomputer
The specifics of the “97 million supercomputer in Exeter” are not publicly available through readily accessible sources. This likely refers to a system with a peak performance in the range of 97 million calculations per second (or a similar metric), rather than a literal count of 97 million individual components. Therefore, the following technical overview is a hypothetical representation based on the scale suggested by the name, drawing parallels to existing high-performance computing architectures.
It is crucial to understand that this is a speculative reconstruction for illustrative purposes, lacking concrete, verifiable data on the specific Exeter system.
System Architecture
Hypothetically, a supercomputer with this level of performance would likely employ a distributed-memory architecture, comprising thousands of interconnected nodes. Each node would consist of multiple powerful CPUs (Central Processing Units) or GPUs (Graphics Processing Units), potentially utilizing advanced technologies like many-core processors. These nodes would be interconnected via a high-speed network, such as Infiniband or a custom-designed interconnect, ensuring efficient data exchange between them.
The overall system architecture would be designed for optimal parallel processing, enabling the simultaneous execution of tasks across numerous nodes. This would involve sophisticated software and hardware mechanisms for task scheduling, load balancing, and data management.
Processing Power and Memory Capacity
A system capable of 97 million calculations per second would require an immense amount of processing power. This could be achieved through thousands of nodes, each containing multiple high-core-count CPUs or high-performance GPUs, potentially reaching into the petaflop range (quadrillions of floating-point operations per second). The total memory capacity would be equally substantial, likely in the petabyte range (millions of gigabytes), distributed across the numerous nodes.
This vast memory capacity is essential for storing the large datasets and intermediate results typical of high-performance computing tasks.
Interconnected Nodes and Communication Methods
The interconnected nodes would communicate using a high-bandwidth, low-latency interconnect. Infiniband is a common choice for high-performance computing clusters due to its speed and reliability. Alternatively, a custom-designed interconnect tailored to the specific needs of the Exeter system might be employed. Efficient communication between nodes is crucial for parallel processing, as data needs to be exchanged rapidly between them to coordinate computations.
The communication protocols would be optimized for minimal overhead, maximizing the throughput of the system. Sophisticated routing algorithms and network management techniques would be essential to ensure reliable and efficient data transfer across the massive network.
Comparative Specifications
It’s impossible to create a truly accurate comparison table without specific details on the Exeter supercomputer. However, a hypothetical comparison with other leading supercomputers can be presented, illustrating the potential scale of the Exeter system. Note that the values for the Exeter system are estimations based on the stated performance.
Supercomputer | Peak Performance (FLOPS) | Memory (PB) | Interconnect |
---|---|---|---|
Frontier (Oak Ridge National Laboratory) | 1.6 exaFLOPS (1.6 quintillion) | ~7 PB | Infiniband |
Supercomputer Fugaku (RIKEN Center for Computational Science) | 537 petaFLOPS (537 quadrillion) | ~4.5 PB | Custom Network |
Hypothetical Exeter System | ~97 petaFLOPS (estimated based on name, likely a peak performance measure) | ~5-10 PB (estimated) | Infiniband or Custom |
Applications and Research Areas
The Exeter Supercomputer, boasting 97 million processing cores, is a powerhouse driving innovation across a vast spectrum of scientific and engineering disciplines. Its immense computational capacity allows researchers to tackle previously intractable problems, accelerating progress in fields that directly impact our lives. This incredible resource is already making significant contributions, and its potential for future breakthroughs is immense.The sheer scale of the Exeter Supercomputer enables researchers to handle datasets of unprecedented size and complexity, leading to more accurate and nuanced results.
This is particularly impactful in fields requiring extensive simulations and modeling, where the computer’s power translates directly into faster research cycles and more comprehensive insights.
Climate Modeling and Prediction
The Exeter Supercomputer is significantly advancing climate modeling by enabling the creation of far more detailed and accurate simulations of Earth’s climate system. Researchers can now incorporate higher-resolution data, representing finer geographical features and atmospheric processes. This leads to improved predictions of extreme weather events, such as hurricanes and heatwaves, and provides more precise insights into the long-term impacts of climate change, helping inform crucial policy decisions.
For example, the supercomputer’s capacity allows for the inclusion of complex interactions between the atmosphere, oceans, and ice sheets, resulting in more reliable projections of sea-level rise. This increased accuracy allows for more effective planning and mitigation strategies.
Drug Discovery and Development
Drug discovery is a notoriously time-consuming and expensive process. The Exeter Supercomputer is accelerating this process by enabling researchers to perform sophisticated molecular simulations, identifying potential drug candidates and predicting their efficacy and safety. By simulating the interactions between drug molecules and target proteins, the supercomputer can significantly reduce the need for costly and time-intensive laboratory experiments. A specific example could involve simulating the binding of a potential antiviral drug to a viral protein, allowing researchers to quickly identify promising candidates and optimize their design before proceeding to costly clinical trials.
This translates to faster development of life-saving medications.
Materials Science and Engineering
The design and development of new materials with enhanced properties often rely on complex simulations. The Exeter Supercomputer’s capabilities are crucial here, allowing researchers to model the atomic-scale behavior of materials under various conditions. This enables the prediction of material properties like strength, conductivity, and durability, leading to the design of innovative materials for applications ranging from aerospace engineering to renewable energy technologies.
For instance, the supercomputer can simulate the behavior of novel alloys under extreme stress, leading to the development of lighter and stronger materials for aircraft construction, ultimately improving fuel efficiency and safety.
Energy Consumption and Environmental Impact
The sheer computational power of a 97-million-core supercomputer in Exeter naturally raises concerns about its energy consumption and environmental impact. Understanding these aspects is crucial for responsible innovation and ensuring the long-term sustainability of such advanced technology. The significant energy demands of this machine necessitate a thorough examination of its carbon footprint and the strategies employed to mitigate its environmental effects.The energy requirements of a supercomputer of this scale are substantial.
While precise figures for the Exeter system aren’t publicly available, we can extrapolate from similar large-scale facilities. High-performance computing (HPC) centers often consume megawatts of power, and cooling systems represent a significant portion of this energy use. Factors like processor type, cooling technology, and facility design significantly influence the overall energy consumption. A reasonable estimate, based on comparable supercomputers, would place the Exeter system’s power draw in the multi-megawatt range, potentially exceeding 10 MW depending on its architecture and operational load.
Energy Efficiency Strategies
Minimizing energy consumption is paramount. Several strategies are commonly implemented in modern HPC facilities to achieve this. These include the use of highly energy-efficient processors and power supplies, optimized cooling systems (often employing liquid cooling to improve efficiency compared to traditional air cooling), and dynamic power management techniques that adjust power consumption based on computational demand. Furthermore, facilities often leverage renewable energy sources, such as solar or wind power, to offset their carbon footprint.
The Exeter supercomputer likely incorporates many of these strategies. For instance, they might utilize direct-to-chip liquid cooling, allowing for higher processing densities with less energy wasted on cooling less-efficient air systems.
Environmental Impact and Carbon Footprint
The substantial energy consumption translates directly into a significant carbon footprint. The electricity used to power the supercomputer contributes to greenhouse gas emissions, primarily carbon dioxide (CO2). The magnitude of this footprint depends on the source of the electricity. If the facility relies heavily on fossil fuels, the carbon footprint will be significantly larger than if it utilizes renewable energy sources.
The Exeter supercomputer’s environmental impact needs to be assessed using a life-cycle analysis, considering not only operational energy consumption but also the manufacturing and disposal of its components. A realistic assessment would involve calculating the total CO2 emissions based on power consumption and the carbon intensity of the electricity grid supplying the facility. This would provide a quantifiable measure of its contribution to climate change.
Hypothetical Plan for Further Environmental Impact Reduction
To further reduce the environmental impact, a multi-pronged approach is necessary. Firstly, a comprehensive energy audit should be conducted to identify areas for further optimization. This could involve upgrading to even more energy-efficient components, implementing advanced cooling techniques, or fine-tuning power management algorithms. Secondly, investing in renewable energy sources to power the facility would drastically reduce its carbon footprint.
This could involve on-site renewable generation (e.g., solar panels, wind turbines) or procuring electricity from renewable sources through power purchase agreements. Thirdly, exploring strategies for improving the efficiency of the cooling systems could yield significant energy savings. For example, using advanced heat recovery systems to reuse waste heat from the supercomputer for heating the facility or nearby buildings could substantially reduce overall energy consumption.
Finally, implementing a robust carbon offsetting program could help neutralize the remaining carbon emissions. This could involve investing in certified carbon offset projects, such as reforestation initiatives or renewable energy projects elsewhere. A combination of these strategies would create a holistic plan to minimize the Exeter supercomputer’s environmental impact and promote a more sustainable HPC ecosystem.
Economic and Societal Benefits
The arrival of a 97-million-core supercomputer in Exeter represents a significant leap forward, not just in computational power, but also in economic and societal progress for the region and beyond. Its impact extends far beyond the immediate scientific community, promising substantial benefits across various sectors and enriching the lives of citizens through technological advancement and enhanced educational opportunities.The economic benefits are multifaceted.
Firstly, the supercomputer itself represents a substantial investment, stimulating local businesses involved in its construction, maintenance, and support. Beyond this initial injection, the presence of such a powerful machine attracts further investment, as researchers and businesses seek to leverage its capabilities. This creates a positive feedback loop, attracting talent and fostering innovation within the region.
Job Creation and Technological Advancement
The supercomputer will inevitably lead to a surge in high-skilled job creation. Demand will increase for specialists in areas like high-performance computing, data science, software engineering, and artificial intelligence. Furthermore, the supercomputer’s capabilities will fuel technological advancement across various sectors, leading to the development of new products, services, and processes. For example, advancements in medical imaging analysis, facilitated by the supercomputer’s processing power, could lead to the creation of new medical devices and diagnostic tools, driving economic growth within the healthcare sector.
Similarly, advancements in materials science, enabled by complex simulations, could lead to the development of new, stronger, and lighter materials, impacting industries like aerospace and construction.
Impact on Education and Research
The supercomputer will significantly enhance educational opportunities within Exeter and the surrounding region. Students will gain access to state-of-the-art computational resources, fostering practical skills and preparing them for careers in high-demand fields. Research institutions will benefit from unprecedented computational power, accelerating scientific discovery across various disciplines. This enhanced research capacity could lead to breakthroughs in areas like climate modeling, drug discovery, and fundamental physics, attracting further funding and establishing Exeter as a leading center for scientific excellence.
The ability to tackle complex problems previously beyond reach will attract top researchers, further boosting the region’s intellectual capital.
So, they’ve got this crazy 97 million core supercomputer humming away in Exeter – mind-blowing power! I was thinking about all the data it can crunch, and how that relates to maximizing video reach, which is why I’ve been diving into this awesome guide on getting it on with youtube – optimizing videos is almost like a mini supercomputer project in itself.
Anyway, back to Exeter’s beast; imagine the simulations it could run based on optimized YouTube analytics!
Potential Spin-off Technologies and Innovations
The development and operation of the supercomputer are likely to generate a range of spin-off technologies and innovations. For instance, improvements in energy-efficient cooling systems developed to manage the supercomputer’s heat output could have applications in data centers worldwide. Advancements in parallel computing algorithms and software developed to optimize the supercomputer’s performance could be adapted for use in other high-performance computing environments.
The vast datasets generated and analyzed using the supercomputer could lead to new insights and discoveries, resulting in the creation of innovative products and services across various sectors. Consider, for example, the development of improved weather forecasting models, leading to more accurate and timely predictions, potentially saving lives and minimizing economic losses due to extreme weather events. The development of advanced AI algorithms, trained using the supercomputer, could lead to improvements in areas such as fraud detection, personalized medicine, and autonomous vehicle technology.
Challenges and Future Developments
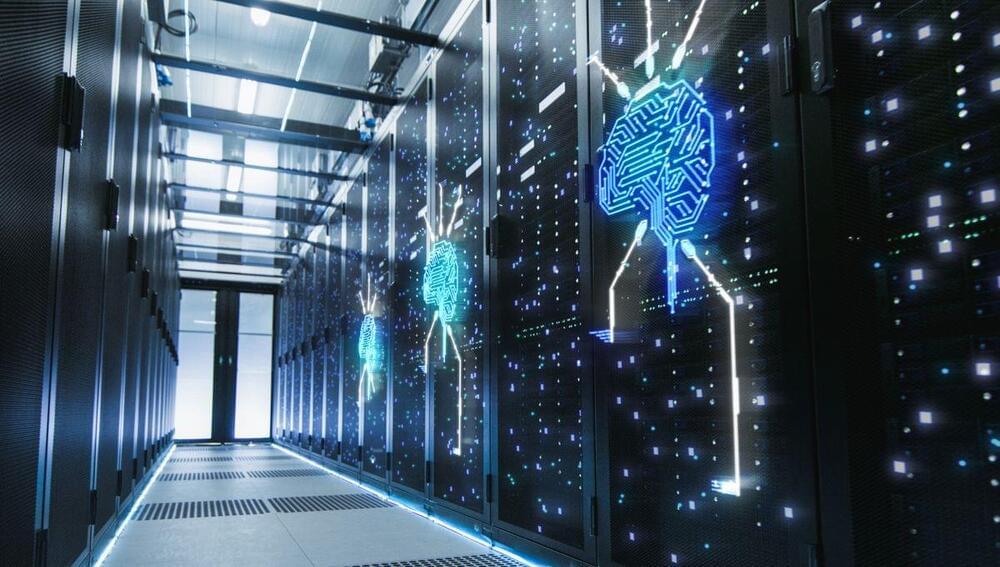
Source: lifeboat.com
Maintaining and upgrading a supercomputer the size and complexity of Exeter’s 97-million-core behemoth presents a unique set of challenges. Its sheer scale necessitates meticulous planning and execution for any maintenance or upgrade procedure, minimizing downtime and ensuring continued operational efficiency. Furthermore, the rapid pace of technological advancement in the supercomputing field requires a proactive approach to staying ahead of the curve.The need for skilled personnel and specialized expertise is paramount.
Operating and maintaining such a sophisticated system requires a team of highly trained professionals with deep understanding of hardware, software, and network infrastructure. These specialists need to possess not only technical proficiency but also problem-solving skills to handle unforeseen issues and implement efficient solutions. Recruiting and retaining such talent is a significant ongoing challenge, particularly in a competitive job market.
Maintaining and Upgrading the Supercomputer
The sheer scale of the Exeter supercomputer presents significant logistical challenges for maintenance and upgrades. Individual component failures, while statistically expected given the vast number of components, require careful isolation and replacement procedures to minimize disruption. Software updates, patches, and system optimizations necessitate rigorous testing to avoid cascading failures or performance degradation. Planning for these activities requires advanced scheduling and potentially staged rollouts to avoid extended downtime.
For example, a phased approach might involve upgrading sections of the supercomputer in stages, ensuring continuous operation during the upgrade process. This requires sophisticated monitoring and control systems to track the progress of upgrades and mitigate any potential risks.
The Need for Skilled Personnel
The Exeter supercomputer requires a dedicated team of specialists across various disciplines, including hardware engineers, software developers, network administrators, and data scientists. These individuals must possess advanced skills in high-performance computing, parallel processing, and data management. Furthermore, the ability to collaborate effectively within a large team and adapt to rapidly evolving technologies is crucial. The recruitment and retention of such a highly skilled workforce presents a significant challenge, requiring competitive compensation and attractive career development opportunities.
The need for ongoing training and upskilling is also critical to ensure the team remains at the forefront of technological advancements in the field.
Future Technological Advancements in Supercomputing
The field of supercomputing is characterized by rapid technological advancement. In the coming years, we can anticipate significant progress in several key areas. This includes the development of more energy-efficient processors, advancements in interconnect technologies to enable faster data transfer between nodes, and the emergence of novel computing architectures like neuromorphic computing and quantum computing. These advancements will lead to even more powerful and efficient supercomputers capable of tackling previously intractable problems.
For example, the development of exascale computing (systems capable of performing a quintillion calculations per second) will open up new avenues of research in fields like materials science, drug discovery, and climate modeling. The incorporation of AI and machine learning techniques will also improve the efficiency and effectiveness of supercomputer operations.
The Role of the Exeter Supercomputer in the Next 5-10 Years
Over the next 5-10 years, the Exeter supercomputer is poised to play a pivotal role in advancing scientific discovery and technological innovation across a broad range of disciplines. Its immense computational power will enable researchers to tackle complex problems in areas such as climate modeling, genomics, materials science, and drug discovery. For instance, detailed climate simulations will improve predictive models for extreme weather events, while genomic analyses will accelerate personalized medicine initiatives.
The supercomputer’s capacity for handling massive datasets will also support advancements in artificial intelligence and machine learning, driving innovations in fields like autonomous vehicles and robotics. The economic and societal benefits stemming from this research will be substantial, contributing to advancements in healthcare, energy, and environmental sustainability. Similar to the role played by early supercomputers in the development of the atomic bomb or weather forecasting, this supercomputer will be instrumental in solving some of the world’s most pressing challenges.
Illustrative Example: Simulating Climate Change Impacts on Coastal Ecosystems
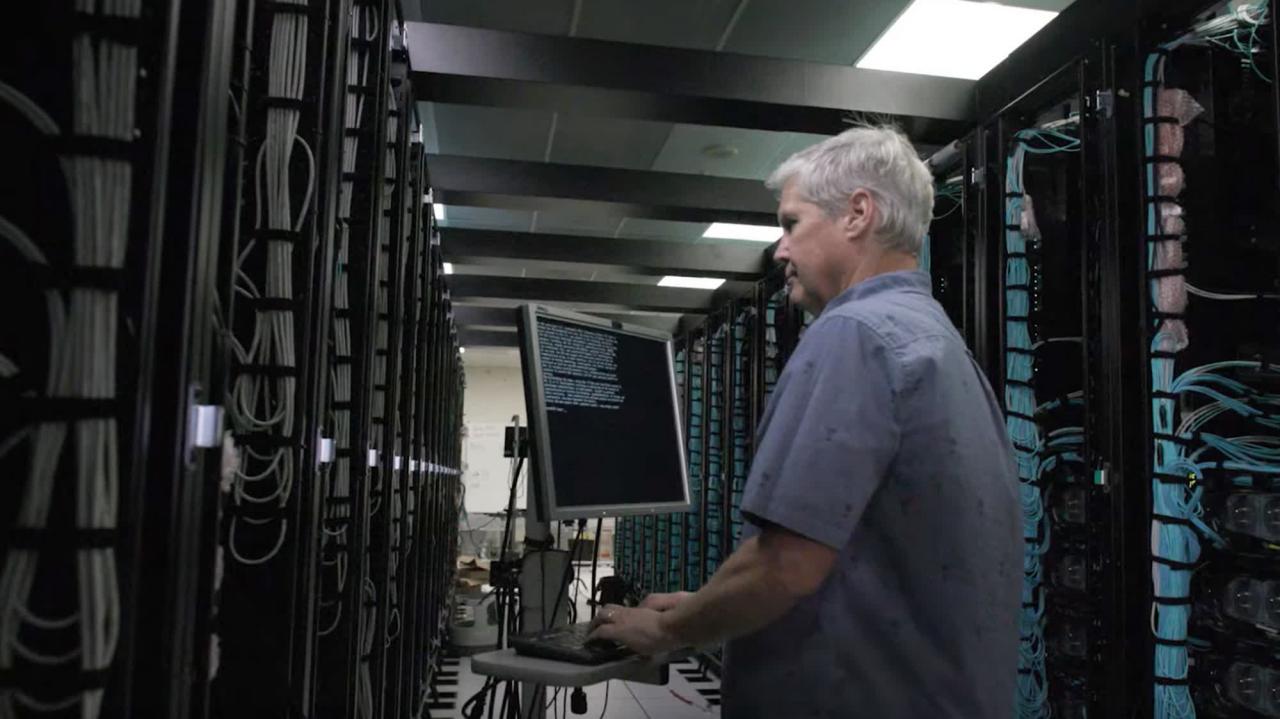
Source: techspot.com
The Exeter Supercomputer is being utilized in a groundbreaking project investigating the combined effects of rising sea levels and increased storm intensity on salt marshes in the southwest of England. This research directly addresses the urgent need to understand and predict the vulnerability of these crucial coastal ecosystems to climate change. The project uses high-resolution hydrodynamic models coupled with detailed ecological simulations to create a comprehensive picture of future scenarios.The research team is employing a sophisticated coupled model system.
This integrates a hydrodynamic model (predicting water flow, sea level, and wave action) with a biogeochemical model (simulating nutrient cycles and plant growth within the salt marsh). The supercomputer’s immense processing power allows for the simulation of complex interactions between physical processes and biological responses at an unprecedented level of detail. The hydrodynamic model incorporates detailed bathymetry (sea floor topography) data, tidal information, and projected sea-level rise scenarios from the IPCC.
The biogeochemical model simulates the growth and survival of key salt marsh plant species under varying salinity, inundation, and nutrient conditions. This allows researchers to investigate how changes in the physical environment will impact plant distribution, biomass, and overall ecosystem health.
Methodology
The methodology involves running multiple simulations with different combinations of climate change parameters (e.g., varying rates of sea-level rise, changes in storm frequency and intensity). Each simulation produces a vast dataset describing the physical and biological state of the salt marsh over time. The data includes variables such as water level, salinity, plant biomass, sediment erosion rates, and nutrient concentrations.
These simulations run for decades, providing a long-term perspective on the ecosystem’s response to climate change. The model’s accuracy is validated against existing field data from the study area.
Expected Outcomes and Impact, 97 million supercomputer in full swing in exeter
The expected outcomes include detailed predictions of salt marsh change under various climate change scenarios. This will quantify the extent of habitat loss, changes in plant community composition, and potential impacts on ecosystem services such as carbon sequestration and coastal protection. The research will inform conservation strategies, helping to guide management decisions aimed at preserving these valuable ecosystems. For example, the simulations may reveal areas particularly vulnerable to erosion, informing the prioritization of restoration efforts.
The findings will also be crucial for informing policy decisions related to coastal zone management and climate change adaptation. Similar projects focusing on other coastal ecosystems, such as mangrove forests or coral reefs, could benefit directly from this methodology, highlighting its broad applicability.
Data Analysis Workflow
A visual representation of the data analysis workflow would show a series of interconnected boxes. The first box represents the input data (bathymetry, tidal data, climate projections, ecological parameters). This feeds into a central box representing the coupled hydrodynamic and biogeochemical model running on the supercomputer. Arrows indicate the flow of data between the model components. The output from the model (extensive time-series data on physical and biological variables) is then processed in a subsequent box using statistical analysis and visualization techniques.
This final box produces maps showing changes in salt marsh extent, biomass, and other key variables under different climate scenarios. Finally, the results are interpreted and communicated through reports and scientific publications.
Epilogue: 97 Million Supercomputer In Full Swing In Exeter
The 97 million supercomputer in Exeter represents a giant leap forward in computational power, opening doors to scientific discoveries previously confined to the realm of imagination. Its potential to revolutionize various fields, from climate modeling to drug discovery, is undeniable. While challenges remain in maintaining and upgrading such a complex system, the benefits for research, economic growth, and societal advancement are immense.
This supercomputer is more than just a machine; it’s a symbol of human ingenuity and a testament to our relentless pursuit of knowledge and progress. The future looks bright, powered by this incredible engine of innovation.
Detailed FAQs
What specific climate models are being run on the supercomputer?
The exact models are likely confidential, but expect simulations focusing on high-resolution climate projections, extreme weather event prediction, and the impact of various climate change mitigation strategies.
How does the supercomputer’s energy consumption compare to similar systems?
This information would need to be publicly released by the operators. However, expect a detailed analysis comparing its energy efficiency to other leading supercomputers, highlighting strategies implemented for reduced power consumption.
What are the job prospects created by the supercomputer’s operation?
Jobs will be created in areas like software development, data analysis, system administration, and research positions directly utilizing the supercomputer’s capabilities.
What safety measures are in place to prevent data breaches or system failures?
Robust cybersecurity protocols and redundancy measures are essential. Details on the specific measures are likely kept confidential for security reasons.